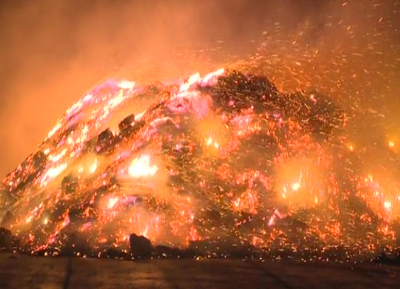
Overview
This unit starts by addressing a common student misconception: that energy is released when bonds break. The first lab focuses on bonds breaking where students will discover that energy is actually required for bonds to break. Students are then provided with a microscopic view of the bonds breaking process so they can explain why energy is absorbed rather than released when bonds break. An exothermic synthesis reaction is demonstrated for students next so they understand that energy is released when bonds form. Students put the two concepts of bond breaking and bond forming together in order to explain overall chemical reactions as endothermic or exothermic. Calorimetry will be employed to measure the energy absorbed or released in a chemical reaction. Students will utilize a calorimetry simulation prior to completing the physical lab so they fully understand what is occurring on a microscopic scale throughout the process and why they are being asked to perform certain calculations to calculate energy.
The second half of the unit introduces students to covalent bonding, including single, double, and triple bonds so students begin to understand that all chemical bonds do not absorb or release the same amount of energy. Finally, students will utilize bond enthalpy tables to calculate the enthalpy of formation of a compound and then the enthalpy of complete chemical reactions.
Gapless Explanation of Anchoring Phenomenon
Spontaneous combustion of a compost pile is only possible if enough heat energy is released by the overall chemical reaction that is occurring within the pile. Organisms within the pile are breaking down the organic matter via cellular respiration. Glucose molecules in the presence of oxygen are combusted to form carbon dioxide and water. The overall reaction can be written as: C6H12O6 + 6 O2 → 6 CO2 + 6 H2O.
To determine the amount of energy released in this reaction, the overall chemical reaction needs to be broken down into steps. Chemical reactions can be thought of as requiring two steps: breaking existing bonds of reactants, then forming new bonds of products. Energy must be absorbed to break bonds, while energy is released when bonds are formed. The absorption of energy to break bonds results in a kinetic energy decrease of the surroundings as kinetic energy is transformed to potential energy within the system. The decrease in kinetic energy of the surroundings is equivalent to a decrease in temperature, this is referred to as endothermic. The opposite occurs when bonds are formed; potential energy of the system is transformed into kinetic energy and released to the surroundings resulting in a kinetic energy increase of the surroundings equivalent to a temperature increase. Bonds forming is exothermic. (When the temperature of the system goes down, to maintain equilibrium, energy is transferred from the surroundings back into the system. Conversely, when the temperature of the system increases, that energy will be released into the surroundings, again, to maintain equilibrium.) This explains the individual steps in a chemical reaction, but not how to determine if a reaction will release energy.
The amount of energy absorbed or released in a chemical reaction can be measured experimentally using calorimetry. In calorimetry, the heat exchanged between the reaction (defined as the system) and the surroundings can be calculated based on measuring the temperature change of the surroundings. For many aqueous reactions, a simple coffee-cup calorimeter may be employed. The calorimeter is two nested Styrofoam cups to provide insulation from the laboratory environment. The chemical reaction will be set up to occur in a specifically measured quantity of solution (the surroundings) so that the mass of the solution is known. During the reaction, the heat evolved or absorbed causes a temperature change in the solution which is measured using a temperature probe. If we know the specific heat of the solution (assumed to be that of water), then the heat absorbed by or lost from the solution (qsolution) can be calculated using the following equation:
qsolution = msolution • Cs solution • ∆T
Energy absorbed or released in a chemical reaction can also be determined by the number and type of bonds broken and formed. Qualitatively, we can look at the number of bonds broken in a chemical reaction versus the number of bonds formed. If more bonds are broken than formed, the overall reaction should be endothermic. If more bonds are formed than broken, the reaction should be exothermic. Though this is usually true, for some reactions, the number of bonds broken and formed is equal. These reactions can still be classified as endothermic or exothermic by quantitative means. The eight lessons in this unit end here.
The overall energy unit continues to quantify bond energy. Students will develop a knowledge of single, double, and triple covalent bonds. A working knowledge of valence electrons of main group elements on the periodic table is needed in order to apply that knowledge again here to determine how many valence electrons a compound has and then to determine how many of those electrons will be shared between each atom in the compound. A simple formula can be employed that uses total valence electrons and electrons needed according to the octet rule to determine how many electrons are shared in a given covalent formula. This formula will help determine if and when double or triple covalent bonds are present in a molecule.
The energy absorbed when bonds are broken is subtracted from the energy released when bonds are made. The overall net energy then identifies that reaction as endothermic or exothermic. A positive net energy is energy absorbed into the system, an endothermic reaction, while a negative net energy is energy released from the system which is an exothermic reaction. Though this subtraction method seems simple enough, what is not possible is to stop a reaction mid-way through after bonds are broken and before new ones are formed to measure energy gained or lost in the system. A table of individual bonds energies becomes necessary. Bond energy is the average energy needed to break or form a bond. If every bond in a compound can be identified, then the energy necessary to either break or make the sum of the bonds can be calculated.
Bond energy is the energy needed to break a bond. If the type of bond present and the two atoms that the bond is between is known then a table of bond energies (*values provided in bond energy tables are averages taken over a variety of compounds that contain a specific atom pair, these values tend to vary slightly from one bond energy table to another) can be used to determine the overall bond enthalpy of a reaction. For the combustion of glucose, the following bond energies are needed to determine the enthalpy for the reaction. These bond energies may vary slightly if acquired from a different table.
C–H = 413 kJ/mol
C–O = 358 kJ/mol
O–H = 467 kJ/mol
O=O = 495 kJ/mol
C=O = 799 kJ/mol
Glucose contains 5 C–C, 7 C–H; 5 C–O, 5 O–H bonds, and 1 C=O bond. The 6 O2 molecules contain 6 O=O bonds. The products contain 12 C=O and 12 O–H bonds.
[(5 * 347) + (7 * 413) + (5 * 358) + (5 * 467) + (1 * 799) + (6 * 495)] – [(12 * 799) + (12 * 467)] =
(1735 + 2891 + 1790 + 2335+ 799 + 2970) – (9588 + 5604) =
12520 – 15192 = –2672 kJ/mol
Underlying Lessons
Standards
Next Generation Science Standards
- Physical Science
Computational Thinking in STEM
- Data Practices
- Modeling and Simulation Practices
- Systems Thinking Practices